Mar 4, 2024
The Nuts and Bolts of Combustion Testing
A look at the basics of combustion and combustion-testing procedures for fuel-burning equipment.
By: Brynn Cooksey, CEM
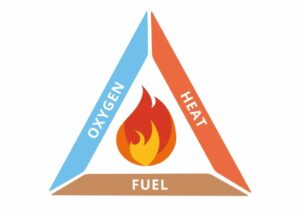
With the heating season in full swing, now is a great time to get back to the basics of combustion performance testing. Combustion performance testing is becoming required in a lot of different areas of the country due to growing concerns of occupant safety, energy consumption and the negative impact of harmful flue gas emissions. Combustion is a process that removes stored energy from fossil fuels. Improper combustion can lead to several problems including, IAQ hazards for occupants, poor equipment performance, equipment failure and wasteful energy usage. Combustion testing is the only proven way that can accurately test and evaluate the performance of fuel-burning equipment. It should be done on every service call and all of the fuel-burning appliances encountered while at a jobsite. Combustion testing saves money and time; prevents callbacks; limits liability; validates manufacturer’s warranty; provides confidence, increased comfort, and safety; increases energy efficiency; reduces harmful flue-gas emissions; and generates revenue.
Definitions
It is important to define several key words related to combustion:
Primary air: The combustion air introduced into a burner that mixes with the fuel before it reaches the burner head.
Secondary air: Combustion air that is externally supplied to a burner flame after combustion has been initiated. Secondary air ensures that there are enough oxygen molecules available for the complete combustion of all of the carbon and hydrogen molecules being supplied to the combustion chamber or zone.
Excess air: The amount of air being supplied to the combustion process over and above what is required for complete combustion. This is air added to the combustion process in addition to secondary air. This is a designed safety measure that is utilized to ensure that there is a surplus of oxygen molecules to achieve complete combustion.
Dilution air: Air that does not participate in the combustion process. Dilution air is added to the flue gases after the combustion process to assist with flue-gas flow out of the stack. It also maintains a high flue-gas temperature to minimize flue-gas condensation.
Tramp air: Air that leaks through improperly seated doors, gaskets, etc. Completely incidental to the combustion process and typically reduces combustion efficiency. Affects combustion performance similar to excess air.
Oxygen O2 (air): The molecule that mixes with the fuel to be burned. Often called the supporter of combustion.
Carbon dioxide (CO2): Byproduct of complete combustion where two molecules of oxygen combine with one molecule of carbon. A high carbon-dioxide reading is desired in a flue-gas sample.
Carbon monoxide (CO): Byproduct of incomplete combustion where one molecules of oxygen combines with one carbon molecule. Carbon-monoxide formation presents potential health and safety conditions as well as an inefficient consumption of fuel.
Flue-gas temperature: The temperature of the flue gases exiting the flue or stack. Indication of combustion performance.
Fuel efficiency: Calculation of the relationship between fuel entering the combustion process and the heat delivered to the fuel burning appliance’s heat-exchanging medium.

Combustion Theory
Combustion is defined as a reaction between a fuel, oxygen, and heat (or source of ignition) that causes the rapid oxidation of a fuel. More simply stated, it means the process of burning a fuel. Combustion is the process in which stored chemical energy in the form of Btu, or heat energy, is converted into another type of energy. The output from this process is heat and light. In order to achieve combustion we need three key components: fuel, oxygen, and heat. To remember this relationship, the combustion requirements are often referred to as the combustion triangle. The first portion of the combustion triangle is the fuel. The fuels used for the combustion process contain stored energy that is utilized for the heat required for homes, businesses and manufacturing processes. Fuels such as natural gas, LP gas and fuel oil all contain hydrocarbons (elements of hydrogen and carbon). It is the hydrogen and carbon elements that actually contain the Btu (heat energy) that we are attempting to liberate. Hydrogen and carbon molecules are mixed with the oxygen molecules to start and sustain the combustion process. Consumers are billed for the fuel they consume on a per-unit basis. The service technician plays a key role by ensuring that the combustion appliance is consuming fuel as efficiently as possible.
Fuel Properties
- Fuels contain the energy that we use for heat and light.
- The fuel being burned must reach a gaseous state or be atomized to start the combustion process.
- Combustion requires proportional amounts of fuel and air to become explosive.
Gaseous fuels — When natural gas is removed from wells in the ground it is referred to as “sour gas” and it contains compounds such as sulfur and other impurities. These compounds are very corrosive and must be removed. This process is known as “sweetening.” When gas has been sweetened, it means it has various amounts of hydrocarbons in it. The gases are processed and separated into wet and dry distinctions. A dry type of gas is natural gas and a wet type of gas is considered to be propane and other gases such as butane.
Natural gas — Natural gas is a fossil fuel that comes from the ground and is the product of decayed organic matter under very large amounts of pressure over an extended period of time. Natural gas contains several gases in small amounts but is more than 95% methane depending on its refinery or utility. On average, natural gas contain about 1,000 Btu per cu ft. Natural gas is lighter than air because it has a lower specific gravity of 0.60, as compared to air at a specific gravity of 1. The chemical composition of natural gas is CH4 (one carbon atom surrounded by four hydrogen atoms). The great advantage of natural gas over other fuels is its relative production, ease of use and transportation. Never assume the fuel-heating value of natural gas. Some utilities supplement natural gas with small amounts of propane or butane. This is done at peak demands to keep the fuel heating value up. Use the natural gas supplier’s published fuel-heating value for all calculations for the most accurate results.
Liquefied petroleum gas (LPG) propane — Propane has some great advantages as a fuel. It stores easily in the liquid form, so it can be easily transported by tank or vessel. Its ease of storage makes it ideal for rural areas where natural gas is not available. Propane has a high energy-heating value of 2,500 Btu per cu ft. This is roughly 2.5 times more than natural gas. In addition, propane burns very clean if provided with adequate amounts of air. The chemical composition of propane is C3H8 (three carbon atoms surrounded by eight hydrogen atoms). Propane has a specific gravity of 1.52, which is heavier than air with a specific gravity of 1. Propane is considerably more expensive than natural gas and is generally utilized in lieu of it.
Fuel oil — Fuel oil is refined from crude oil by a process called cracking. Fuel oil used for heating applications is distilled from crude oil and is then processed with certain additives to give fuel oil its desired heating valve and flow characteristics. Fuel oil, like LP gas, is also used in areas where natural gas is unavailable or expensive. Also, fuel oil is easily stored and handled, which is one of its advantages. Fuel oil applications have many code requirements that must be strictly followed for personnel safety and to prevent property damage by fire. Fuel oil must be atomized prior to its ignition. This promotes the proper mixing of the oxygen and fuel prior to being burned.
Air (oxygen) — The second portion of the combustion triangle is the oxygen delivered by air. In the HVAC industry, the terms air and oxygen are often interchanged, but it is the oxygen that is needed for combustion. Air is composed primarily of nitrogen (79.1%) and oxygen (20.9%). In addition to nitrogen and oxygen, there are several other trace gases that are in the atmosphere that exist in extremely small amounts and are normally not considered when evaluating combustion gases. Because we use the air in our atmosphere for the vehicle for oxygen delivery, we must cope with the presence of nitrogen. Nitrogen absorbs heat, increases the volume of the flue gases and reduces efficiency. The presence of nitrogen makes the combustion process less efficient. The unfortunate truth is that we are stuck with nitrogen and simply ignore its impact.
Heat — The third part of the triangle is the source of ignition, or heat, required to start the combustion process. Once the combustion process is started, and if the fuel and air ratio input remains unchanged, the combustion process is self sustaining or will continue to burn until the fuel or air is removed. When combustion has started, the temperature must remain at or above the ignition temperature or combustion stops. The heat produced by combustion maintains the ignition temperature. The heat from the burning fuel ignites the following air-fuel mixture. Therefore, if we have a combustion-related problem, we have a fuel-to-air ratio problem.
Combustion Performance
Combustion is classified into three main categories:
- Perfect combustion or stoichiometric: The burning of a fuel with the exact fuel-to-air ratio where there is no excess air or carbon monoxide present. This is only accomplished in a laboratory and should not be attempted in the field.
- Complete combustion: The complete burning of all of the fuel molecules with the presence of some excess air. This is what we strive for when combustion testing.
- Incomplete combustion: Incomplete combustion occurs when there is an improper mixture of fuel and air. One of the constituents of the fuel-air mixture is either too high or too low. Incomplete combustion often shows symptoms such as soot, carbon-monoxide formation and reduced efficiency. In addition, incomplete combustion is extremely inefficient. Often times the fuel is not completely burned and still has heating value remaining in it, but it is still billed to customers on a per unit bases.
Some causes for incomplete combustion include: inadequate supply of air; too much fuel; inadequate mixing of air and fuel; flame impingement; too much draft; not enough draft; lack of flame temperature (often seen on startup); equipment damage; competing systems; or improper building pressures.
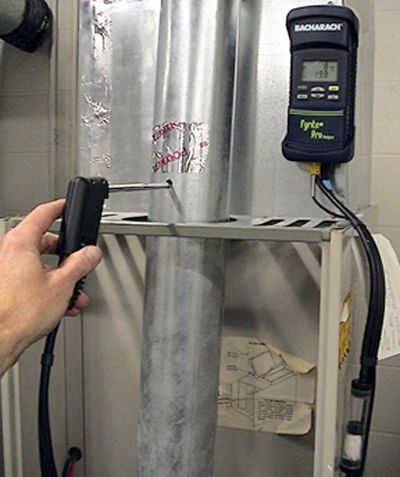
Combustion Testing Procedures
Combustion analyzers are very accurate and sensitive instruments, and should be handled with care. Manufacturer required maintenance and calibration should be adhered to at all times. Manufacturers have different methods for maintenance and calibrations for their different machines, so what may apply to one machine may be different from another. As always, refer to the manufacturer for the specific procedure and steps taken. Most combustion analyzers have sensors that measure the percent of oxygen in a flue-gas sample. Other factors are calculated based on the fuel factors that are known for a particular fuel such as carbon dioxide. This is because there is a relationship that exists between oxygen and carbon dioxide. Oxygen and carbon dioxide are indirectly proportional, meaning that if the value of one of the parameters goes up the other parameter will go in the opposite direction. Other parameters are measured such as carbon monoxide, stack temperature and nitric oxide. Combustion analyzers take a continuous sample of flue gases to quickly report changing burner conditions.
The following are some good rules to live by no matter what combustion-testing device is being used:
- Always obtain an undiluted flue-gas sample. This means sampling flues before barometric dampers or draft diverters. Sampling flues after these draft-control devices will cause the incorrect flue-gas information. Also, if possible, take a sample before any elbows or change of direction. The probe of an analyzer should be in the center of the pipe to get the most accurate sample.
- Only measure combustion gases when the fuel-burning appliance has reached steady state. Some technicians like to associate this with a time function such as 5 or 10 minutes, which is incorrect. Combustion samples should be taken when the parameters stop changing. Whenever a combustion appliance first starts up, combustion is extremely inefficient. This is because the heat exchanger is not warm enough to reflect radiation heat back into the combustion process. Some of the molecules make it through the combustion zones unburned or they may have not even been started in the first place.
- For every action there is a reaction. When making a burner adjustment, you have adjusted the fuel-to-air ratio in some fashion. After the adjustment, the sampling process should restart after waiting for the parameters to stop changing.
- Test, adjust and verify. Reaction to adjustments too quickly may cause the combustion performance to be further off than desired. Also verify the adjustments made with several trials or test of flue-gas samples.
- Never try to achieve perfect combustion. Excess air is an insurance policy for combustion appliances. Adjustments should not be made to completely eliminate excess air.
Recommended Combustion Parameters
When testing combustion equipment, the following questions should be considered: Is there a procedure or guidance for this piece of equipment written in the instructions? Has the unit been commissioned properly and verified by testing? Is the combustion process operating in the correct range? Always follow the equipment manufacturer’s combustion requirements. In lieu of no information available, the following table outlines some industry-acceptable values.
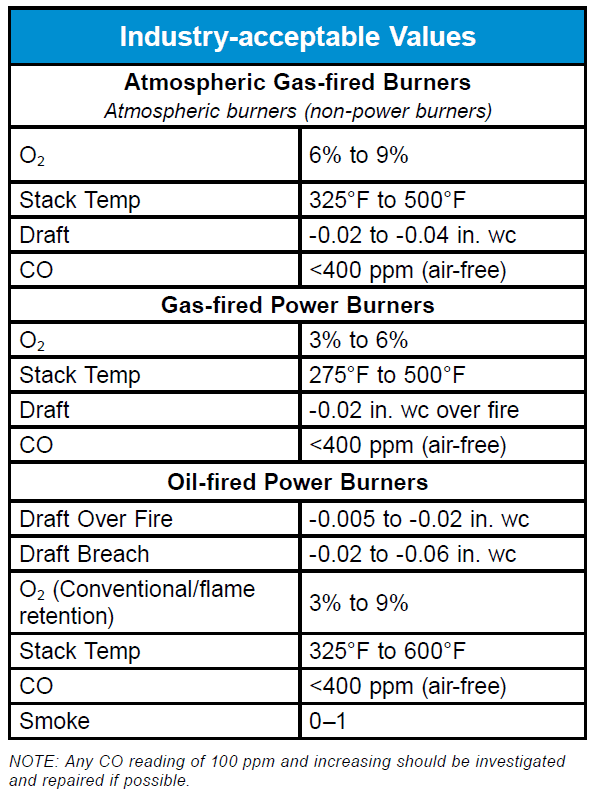
Keep in mind, combustion analysis is only part of the equipment installation, commissioning and servicing procedure. A complete system overview should include: proper system design (sizing and selection); proper air flow; proper fuel pressure; proper draft; combustion air; ventilation air; and functioning limit and safety controls.
Brynn Cooksey will be speaking at BPA’s upcoming National Home Performance Conference & Trade Show taking place in Minneapolis, Minnesota from April 8-11. His session, HVACR System Performance – Combustion Analysis and Fuel Efficiency, will take place Monday, April 8 from 3:30 to 5:00 pm.
Article reprinted with permission from RSES Journal (www.rsesjournal.com).